HERA’s latest study indicates that with the right design choices, carbon emissions can be cut by more than 50% – starting today.
A HERA-led study indicates that readily achieving our national carbon reduction targets in the built environment is not just possible – it’s within our grasp through clever design choices. The building research levy (through BRANZ) and heavy engineering research levy (through HERA) funded study is titled “Circular design for a changing environment: a design framework to reduce construction waste, lifecycle embodied carbon, and to enhance the circular economy for construction materials, with a pilot for low-rise buildings. The study aims to develop practical guidance to significantly reduce carbon emissions in construction.
Low carbon circular design strategies
Imagine being able to halve the carbon in low-rise commercial buildings you are designing – just through some simple changes. That’s the potential of implementing HERA’s low carbon circular design hierarchy (refer to Figure 1) and specific design guidance for low-rise commercial buildings (to be released shortly).
These documents are intended to be a useful resource for designers, specifiers, engineers and other practitioners in the sector with an interest in either low carbon or circular design. Think of it like the waste hierarchy (which gives general guidance on waste reduction) but for carbon! The specific guidance will enable the design of low-rise commercial buildings that are not only low carbon but also support the circular economy for building materials and systems.
The framework and specific design guidance covers both new builds and re-use of existing buildings. As part of the framework, we’ve developed a low carbon circular design hierarchy (“the Hierarchy”) as shown in Figure 1.
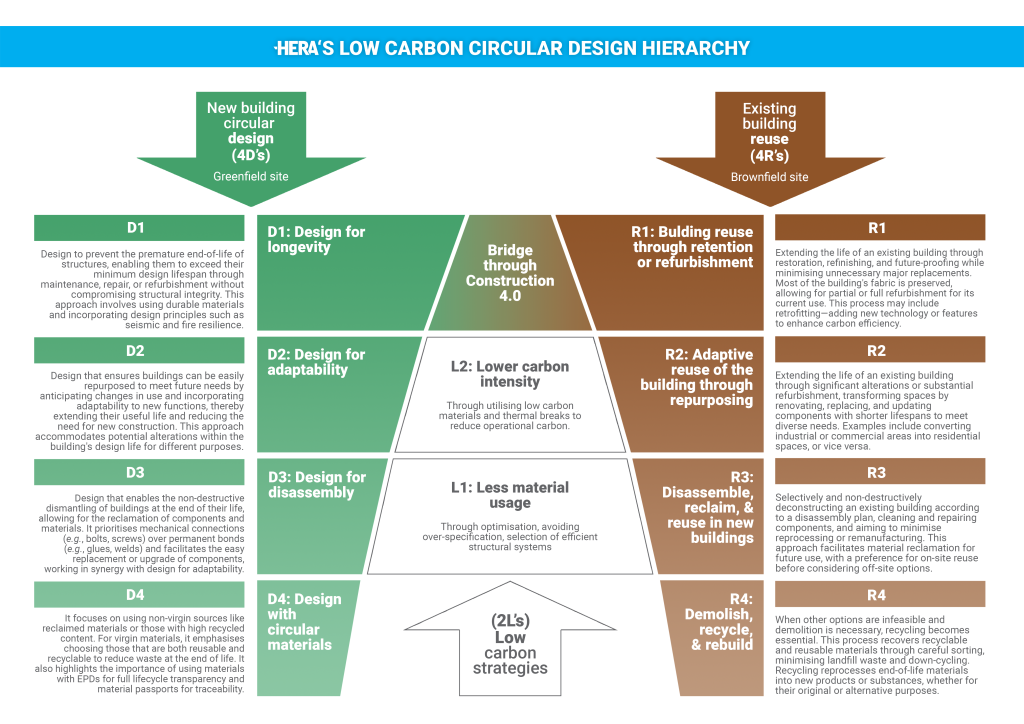
Case study: simple swaps, big impact!
To put the Hierarchy to the test to assess the impact of using different construction materials and details, we looked at six low-rise buildings, each assessed using Life Cycle Assessment (LCA) analysis.
Among these, an existing commercial office building in Christchurch from circa 2014, designated as Building 3 (“B3”), was selected as the reference building, and was chosen because its Cradle-to-Cradle embodied emissions were closest to the average among the nominated buildings – making it the best representative benchmark for the study. This building had a conventional steel-concrete composite flooring system, using a metal decking floor system, and as its main seismic resistant system concrete shear walls in one direction and steel moment resisting frames (MRF) in the other direction were used. The superstructure was supported on a raft foundation (see Figure 3).
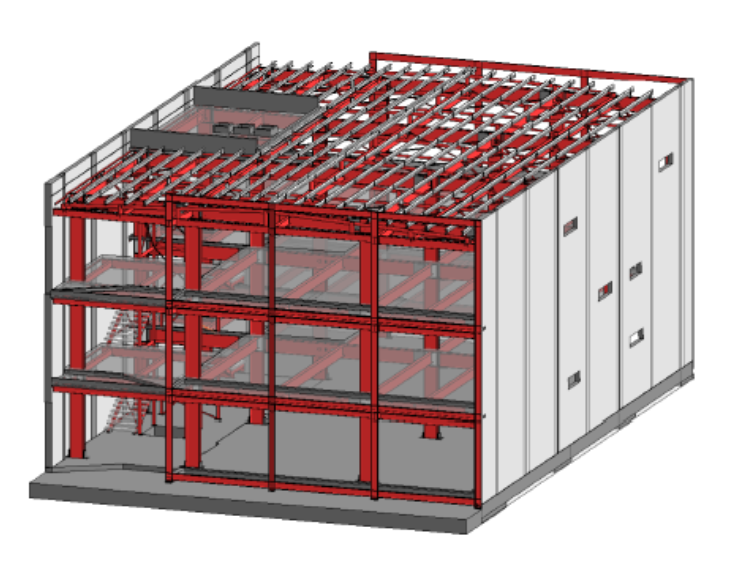
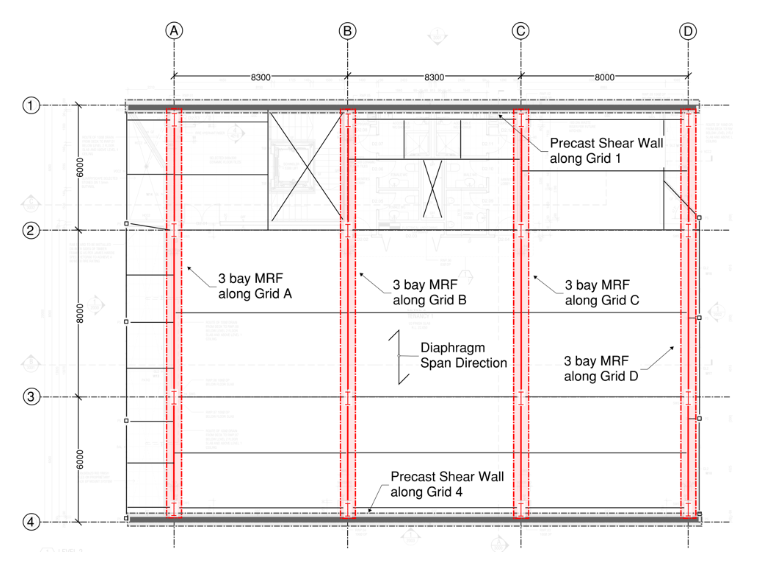
The details of the underlying LCA work, including the LCA method, assumptions, functional units, inventory analysis and similar, are to be published in the forthcoming SESOC journal article titled “Towards proposing and piloting a framework for low carbon design: an introduction”. HERA also plans to publish the specific guidance for low-rise commercial buildings shortly.
Six designs – six pathways to less carbon
We explored several design variations, with each variation showing us just how much carbon we could cut with small but strategic design choices.
In summary, the case study design variations compared against the “As built” (Option 1) reference included:
- Option 1: B3, our baseline reference building.
A conventional steel/concrete composite flooring with steel moment resisting frame (MRF) in one direction and Concrete shear wall in the orthogonal direction as lateral resisting systems, using 2014 design and construction best practices.
- Option 1a: B3, our baseline reference building with low carbon steel.
- Option 1b: B3, our baseline reference building with low carbon steel and concrete.
- Option 1c: B3, our baseline reference building with low carbon concrete.
- Option 2: B3_Con
As Option 1 but replacing the steel moment resisting frames with reinforced concrete frames, resulting in an all-concrete solution, using 2020 design and construction best practices.
- Option 2c: B3_Con with low carbon concrete
- Option 3: B3_Tim&St&Con
As Option 1 but replacing the steel-concrete composite flooring with a steel-timber hybrid flooring system.
- Option 4: B3_St-EBF
As Option 1 but replacing the concrete shear walls with Eccentrically Braced Frames (EBFs), whilst keeping the remainder of the reference building as is.
- Option 4a: B3_St-EBF with low carbon steel.
- Option 4b: B3_St-EBF with low carbon concrete and steel.
- Option 5: B3_St&Tim-EBF
As option 3 but replacing the concrete shear walls with EBFs.
- Option 5: B3_St&Tim-EBF with low carbon steel.
- Option 6: B3_MassTim
As Option 1, but replacing the steel MRFs with timber frames, replacing the concrete shear walls with a steel-concrete stairwell core, using 2020 design and construction best practices. This design of this option was supplied by PTL Structural & Fire, on behalf of Timber Unlimited.
Tables 1 and 2 below show the significant potential for carbon reduction, based on our case study, for carbon reductions using the Hierarchy and specific design guidance for low rise commercial buildings.
Options | Fossil Carbon | Whole-of-life
Biogenic Carbon |
||||
A | C | D | Cradle-to-Cradle
(A-D) |
Difference
Relative to Option 1
|
||
1 | 377 | 14 | -143 | 248 | – | 0 |
1a | 187 | 17 | -8 | 196 | -21% | 0 |
1b | 166 | 17 | -8 | 175 | -29% | 0 |
1c | 356 | 14 | -143 | 227 | -8% | 0 |
2 | 408 | 22 | -51 | 379 | 53% | -13 |
2c | 370 | 22 | -51 | 341 | -10% (relative to option 2) |
-13 |
3 | 362 | 26 | -150 | 238 | -4% | -93 |
4 | 306 | 10 | -155 | 161 | -35% | 0 |
4a | 90 | 13 | -2 | 101 | -59% | 0 |
4b | 81 | 13 | -2 | 92 | -63% | 0 |
5 | 250 | 20 | -140 | 130 | -48% | -93 |
5a | 52 | 23 | -1 | 74 | -70% | -93 |
6 | 48 | 22 | -10 | 60 | -76% | -182 |
*The Negative sign indicates a reduction relative to the reference. |
Table 1: Embodied Carbon of superstructure with potential carbon removals (kgCO2eq/m2).
No | Strategy | Solution | Superstructure carbon emission (kgCO2eq/m2) | ||||||
Life cycle modulus | Total (non-biogenic) | Biogenic | Carbon reduction % | Cumulative carbon reduction % | |||||
A | C | D | |||||||
Reference Building | 377 | 14 | -143 | 248 | 0 | N.A | |||
1 | Design for disassembly | Reversible connection in flooring systems | 377 | 13 | -153 | 237 | 0 | 5 | 5 |
2 | Design for longevity | Seismic resilience (steel frame design) | 377 | 13 | -169 | 221 | 0 | 6 | 11 |
3 | Low Carbon Intensity | Specify Low-carbon concrete (LC-40%) | 356 | 14 | -169 | 201 | 0 | 8 | 19 |
Specify Low-carbon structural steel | 166 | 17 | -33 | 150 | 0 | 21 | 40 | ||
Specify Low-carbon reinforcing rebs | 80 | 17 | 9 | 106 | 0 | 17 | 57 |
Table 2. Impacts of three low carbon circular design strategies from the Hierarchy
Clever design can significantly cut carbon
Based on these results, our case study indicates significant potential to substantially reduce carbon in our built environment through smart design and optimal use of materials. Steel, timber and concrete each have a role to play.
One important takeaway from our project is the need for caution when interpreting LCA studies, especially around making claims about relative material performance. A key finding was the importance of ensuring that LCA tools are kept up-to-date with low carbon material options (such as steel and concrete) which are already available in our market.
Unfortunately, we found that many LCA tools currently lack the most up-to-date low carbon options with associated Environmental Product Declarations to reliably base global warming potential values. This means that some LCA studies, using outdated tools will not reflect the benefits arising from low carbon options. As a result, caution is required when claims are being made of relative material performance as this can erroneously skew comparisons significantly in favour of one material over another.
Key considerations for practitioners interested in low carbon circular design
- Clever design can lead to big carbon savings: widespread adoption of our Hierarchy and specific guidance for low-rise buildings, would make meaningful carbon reductions possible.
- Cradle to cradle thinking matters! In addition to upfront carbon assessment, adoption of a cradle-to-cradle approach (Modules A to D) benefits beyond the building’s life. It also enables separate reporting of the whole-of-life sequestration potential of the building option.
- Explore your options. When assessing different design solutions, such as the selection of a seismic resisting systems and flooring systems, consider various alternatives to identify the optimal low carbon solution.
- Be careful about claims that one material is better than the other - maximising the advantages of steel, concrete, and timber through clever design and specification, will assist the sector to meet our national carbon reduction targets while optimising performance, resilience and cost-efficiency.
- New low-carbon materials exist! Low carbon steel and concrete alternatives already exist in our market and may offer significant carbon reductions alongside the other associated performance or cost benefits.
- Updates to LCA tools should be comprehensive. They should reflect the broad range of products being used and available within our market and capture the latest EPDs. This may require creation of a formal process of review and engagement with the sector to ensure this is achievable without great effort.
Resources available to get you started!
HERA will shortly publish the specific guidance for low rise commercial buildings freely on our Ngākopa Construction 4.0 Hub and we have plans to expand this work to include more case studies and insights. We’ll also explore other building typologies, planetary accounting considerations and costs associated with various design options.
If you’d like a poster version of the Low Carbon Circular Design Hierarchy for your office, please get in touch with our Customer Experience Manager, Rebecca Symonds.
HERA also has a podcast relating to the project that you can access, and we are happy to do free in-house training for any practitioners where minimum numbers can be guaranteed. Please get in touch with our CEO Troy Coyle to discuss further. HERA also has plans to expand the design guidance out to include mid and high-rise construction and bridges, as well as consider cost impacts and planetary accounting.
About
Dr Troy Coyle brings more than 20 years’ experience in innovation management across a range of industries including materials science, medical radiation physics, biotechnology, sustainable building products, renewable energy and steel. She is a scientist with a PhD (University of NSW) with training in journalism and communications. She is the CEO of HERA – an impact led independent research association, focused on heavy engineering and its applications in manufacturing and construction.
mutu | ends